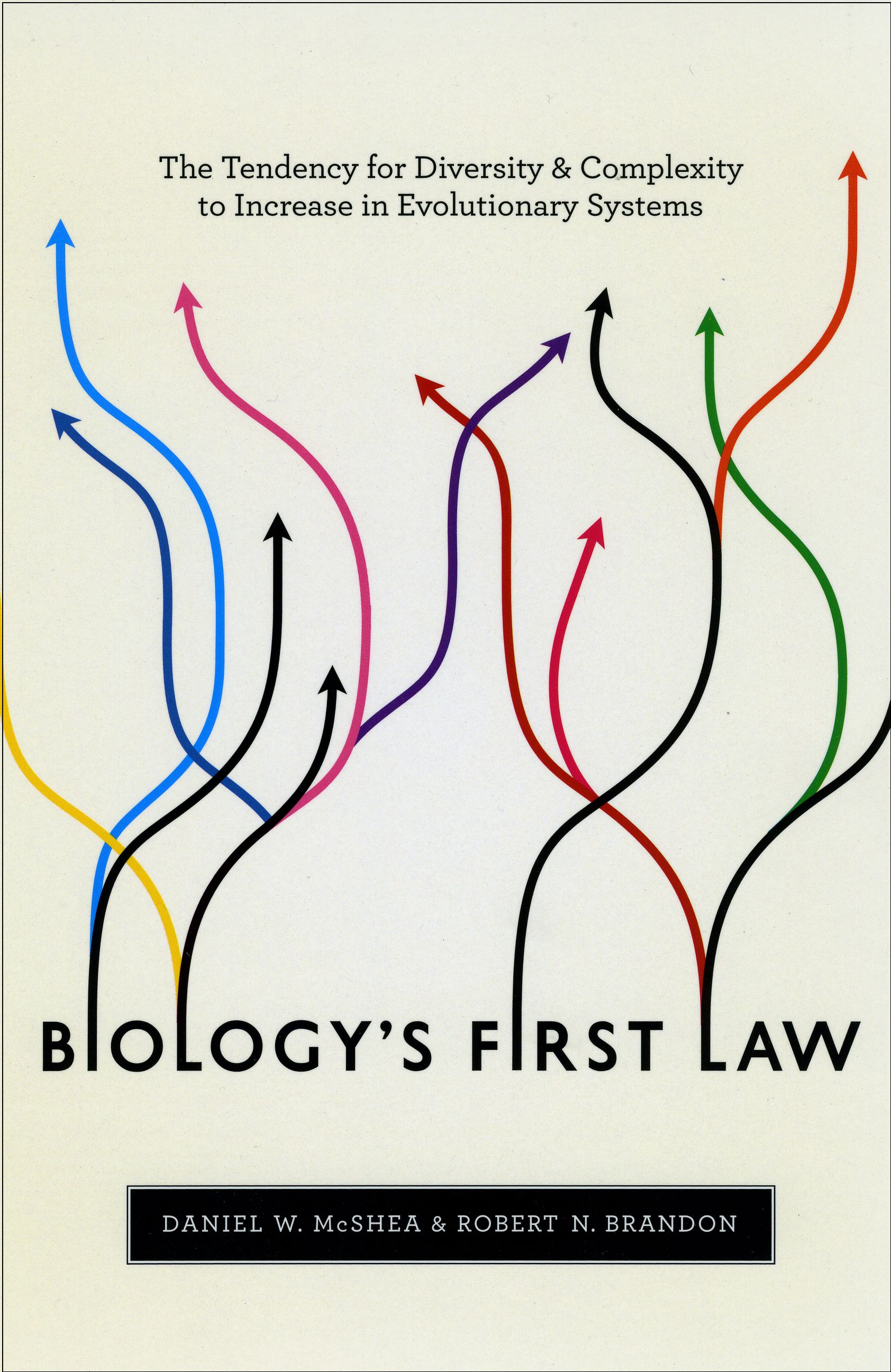
From the current issue of Scientific American, science writer Carl Zimmer offers an extensive overview of the complexity of evolutionary processes - and the tendency for complexity and diversity to increase in evolutionary systems.
A group of Canadian biologists, including Michael Gray of Dalhousie University in Halifax, proposed that some mutations might give rise to complex structures "without going through a series of intermediates that are each selected for their help in adapting an organism to its environment. They dubbed this process constructive neutral evolution."
Two of the authors featured in this piece, Daniel W. McShea and Robert N. Brandon (authors of Biology's First Law: The Tendency for Diversity and Complexity to Increase in Evolutionary Systems, 2010), have proposed (in that book) something called the zero force evolutionary law (ZFEL).
ZFEL says (roughly) that when there are no evolutionary forces acting on a population, the population’s complexity (i.e., how diverse its member organisms are) will increase.There is some really interesting research presented here - and Zimmer is one of the best science writers around.
The Surprising Origins of Evolutionary Complexity
Scientists are exploring how organisms can evolve elaborate structures without Darwinian selection
By Carl Zimmer | Tuesday, July 16, 2013
LAB-RAISED fruit flies are more complex than wild ones because their sheltered environment allows even disadvantageous mutations to spread. This artist's conception contrasts typical wild fly anatomy (left) with representative mutations that arise in lab flies (right). Image: Cherie Sinnen
Charles Darwin was not yet 30 when he got the basic idea for the theory of evolution. But it wasn't until he turned 50 that he presented his argument to the world. He spent those two decades methodically compiling evidence for his theory and coming up with responses to every skeptical counter-argument he could think of. And the counterargument he anticipated most of all was that the gradual evolutionary process he envisioned could not produce certain complex structures.
Consider the human eye. It is made up of many parts—a retina, a lens, muscles, jelly, and so on—all of which must interact for sight to occur. Damage one part—detach the retina, for instance—and blindness can follow. In fact, the eye functions only if the parts are of the right size and shape to work with one another. If Darwin was right, then the complex eye had evolved from simple precursors. In On the Origin of Species, Darwin wrote that this idea “seems, I freely confess, absurd in the highest possible degree.”
But Darwin could nonetheless see a path to the evolution of complexity. In each generation, individuals varied in their traits. Some variations increased their survival and allowed them to have more offspring. Over generations those advantageous variations would become more common—would, in a word, be “selected.” As new variations emerged and spread, they could gradually tinker with anatomy, producing complex structures.
The human eye, Darwin argued, could have evolved from a simple light-catching patch of tissue of the kind that animals such as flatworms grow today. Natural selection could have turned the patch into a cup that could detect the direction of the light. Then, some added feature would work with the cup to further improve vision, better adapting an organism to its surroundings, and so this intermediate precursor of an eye would be passed down to future generations. And, step-by-step, natural selection could drive this transformation to increased complexity because each intermediate form would provide an advantage over what came before.
Darwin's musings on the origin of complexity have found support in modern biology. Today biologists can probe the eye and other organs in detail at the molecular level, where they find immensely complex proteins joining together to make structures that bear a striking resemblance to portals, conveyor belts and motors. Such intricate systems of proteins can evolve from simpler ones, with natural selection favoring the intermediates along the way.
But recently some scientists and philosophers have suggested that complexity can arise through other routes. Some argue that life has a built-in tendency to become more complex over time. Others maintain that as random mutations arise, complexity emerges as a side effect, even without natural selection to help it along. Complexity, they say, is not purely the result of millions of years of fine-tuning through natural selection—the process that Richard Dawkins famously dubbed “the blind watchmaker.” To some extent, it just happens.
A Sum of Varied Parts
Biologists and philosophers have pondered the evolution of complexity for decades, but according to Daniel W. McShea, a paleobiologist at Duke University, they have been hobbled by vague definitions. “It's not just that they don't know how to put a number on it. They don't know what they mean by the word,” McShea says.
McShea has been contemplating this question for years, working closely with Robert N. Brandon, also at Duke. McShea and Brandon suggest that we look not only at the sheer number of parts making up living things but at the types of parts. Our bodies are made of 10 trillion cells. If they were all of one type, we would be featureless heaps of protoplasm. Instead we have muscle cells, red blood cells, skin cells, and so on. Even a single organ can have many different cell types. The retina, for example, has about 60 different kinds of neurons, each with a distinct task. By this measure, we can say that we humans are, indeed, more complex than an animal such as a sponge, which has perhaps only six cell types.
One advantage of this definition is that you can measure complexity in many ways. Our skeletons have different types of bones, for example, each with a distinctive shape. Even the spine is made up of different types of parts, from the vertebrae in the neck that hold up our head to the ones that support our rib cage.
In their 2010 book Biology's First Law, McShea and Brandon outlined a way that complexity defined in this way could arise. They argued that a bunch of parts that start out more or less the same should differentiate over time. Whenever organisms reproduce, one or more of their genes may mutate. And sometimes these mutations give rise to more types of parts. Once an organism has more parts, those units have an opportunity to become different. After a gene is accidentally copied, the duplicate may pick up mutations that the original does not share. Thus, if you start with a set of identical parts, according to McShea and Brandon, they will tend to become increasingly different from one another. In other words, the organism's complexity will increase.
As complexity arises, it may help an organism survive better or have more offspring. If so, it will be favored by natural selection and spread through the population. Mammals, for example, smell by binding odor molecules to receptors on nerve endings in their nose. These receptor genes have repeatedly duplicated over millions of years. The new copies mutate, allowing mammals to smell a wider range of aromas. Animals that rely heavily on their nose, such as mice and dogs, have more than 1,000 of these receptor genes. On the other hand, complexity can be a burden. Mutations can change the shape of a neck vertebra, for instance, making it hard for the head to turn. Natural selection will keep these mutations from spreading through populations. That is, organisms born with those traits will tend to die before reproducing, thus taking the deleterious traits out of circulation when they go. In these cases, natural selection works against complexity.
Unlike standard evolutionary theory, McShea and Brandon see complexity increasing even in the absence of natural selection. This statement is, they maintain, a fundamental law of biology—perhaps its only one. They have dubbed it the zero-force evolutionary law.
The Fruit-Fly Test
Recently McShea and Leonore Fleming, a graduate student at Duke, put the zero-force evolutionary law to the test. The subjects wereDrosophila flies. For more than a century scientists have reared stocks of the flies to use in experiments. In their laboratory homes, the flies have led a pampered life, provided with a constant supply of food and a steady, warm climate. Their wild relatives, meanwhile, have to contend with starvation, predators, cold and heat. Natural selection is strong among the wild flies, eliminating mutations that make flies unable to cope with their many challenges. In the sheltered environment of the labs, in contrast, natural selection is feeble.
The zero-force evolutionary law makes a clear prediction: over the past century the lab flies should have been less subject to the elimination of disadvantageous mutations and thus should have become more complex than the wild ones.
Fleming and McShea examined the scientific literature for 916 laboratory lines of flies. They made many different measures of complexity in each population. In the journal Evolution & Development, they recently reported that the lab flies were indeed more complex than wild ones. Some of the insects had irregular legs. Others acquired complicated patterns of colors on their wings. The segments of their antennae took on different shapes. Freed from natural selection, flies have reveled in complexity, just as the law predicts.
Although some biologists have endorsed the zero-force evolutionary law, Douglas Erwin, a leading paleontologist at the Smithsonian National Museum of Natural History, thinks it has some serious flaws. “One of its basic assumptions fails,” he argues. According to the law, complexity may increase in the absence of selection. But that would be true only if organisms could actually exist beyond the influence of selection. In the real world, even when they are pampered by the most doting of scientists, Erwin contends, selection still exerts a force. For an animal such as a fly to develop properly, hundreds of genes have to interact in an elaborate choreography, turning one cell into many, giving rise to different organs, and so on. Mutations may disrupt that choreography, preventing the flies from becoming viable adults.
An organism can exist without external selection—without the environment determining who wins and loses in the evolutionary race—but it will still be subject to internal selection, which takes place within organisms. In their new study, McShea and Fleming do not provide evidence for the zero-force evolutionary law, according to Erwin, “because they only consider adult variants.” The researchers did not look at the mutants that died from developmental disorders before reaching maturity, despite being cared for by scientists.
Another objection Erwin and other critics have raised is that McShea and Brandon's version of complexity does not jibe with how most people define the term. After all, an eye does not just have many different parts. Those parts also carry out a task together, and each one has a particular job to do. But McShea and Brandon argue that the kind of complexity that they are examining could lead to complexity of other sorts. “The kind of complexity that we're seeing in this Drosophila population is the foundation for really interesting stuff that selection could get hold of” to build complex structures that function to aid survival, McShea says.
Molecular Complexity
As a paleobiologist, McShea is accustomed to thinking about the kind of complexity he can see in fossils—bones fitting together into a skeleton, for example. But in recent years a number of molecular biologists have independently begun to think much as he does about how complexity emerges.
In the 1990s a group of Canadian biologists started to ponder the fact that mutations often have no effect on an organism at all. These mutations are, in the jargon of evolutionary biology, neutral. The scientists, including Michael Gray of Dalhousie University in Halifax, proposed that the mutations could give rise to complex structures without going through a series of intermediates that are each selected for their help in adapting an organism to its environment. They dubbed this process “constructive neutral evolution.”
Gray has been encouraged by some recent studies that provide compelling evidence for constructive neutral evolution. One of the leaders in this research is Joe Thornton of the University of Oregon. He and his colleagues have found what appears to be an example in the cells of fungi. In fungi, such as a portobello mushroom, cells have to move atoms from one place to another to stay alive. One of the ways they do so is with molecular pumps called vacuolar ATPase complexes. A spinning ring of proteins shuttles atoms from one side of a membrane in the fungus to another. This ring is clearly a complex structure. It contains six protein molecules. Four of the molecules consist of the protein known as Vma3. The fifth is Vma11 and the sixth Vma16. All three types of protein are essential for the ring to spin.
To find out how this complex structure evolved, Thornton and his colleagues compared the proteins with related versions in other organisms, such as animals. (Fungi and animals share a common ancestor that lived around a billion years ago.)
In animals, the vacuolar ATPase complexes also have spinning rings made of six proteins. But those rings are different in one crucial way: instead of having three types of proteins in their rings, they have only two. Each animal ring is made up of five copies of Vma3 and one of Vma16. They have no Vma11. By McShea and Brandon's definition of complexity, fungi are more complex than animals—at least when it comes to their vacuolar ATPase complexes.
The scientists looked closely at the genes encoding the ring proteins. Vma11, the ring protein unique to fungi, turns out to be a close relative of the Vma3 in both animals and fungi. The genes for Vma3 and Vma11 must therefore share a common ancestry. Thornton and his colleagues concluded that early in the evolution of fungi, an ancestral gene for ring proteins was accidentally duplicated. Those two copies then evolved into Vma3 and Vma11.
By comparing the differences in the genes for Vma3 and Vma11, Thornton and his colleagues reconstructed the ancestral gene from which they both evolved. They then used that DNA sequence to create a corresponding protein—in effect, resurrecting an 800-million-year-old protein. The scientists called this protein Anc.3-11—short for ancestor of Vma3 and Vma11. They wondered how the protein ring functioned with this ancestral protein. To find out, they inserted the gene for Anc.3-11 into the DNA of yeast. They also shut down its descendant genes, Vma3 and Vma11. Normally, shutting down the genes for the Vma3 and Vma11 proteins would be fatal because the yeast could no longer make their rings. But Thornton and his co-workers found that the yeast could survive with Anc.3-11 instead. It combined Anc.3-11 with Vma16 to make fully functional rings.
Experiments such as this one allowed the scientists to formulate a hypothesis for how the fungal ring became more complex. Fungi started out with rings made from only two proteins—the same ones found in animals like us. The proteins were versatile, able to bind to themselves or to their partners, joining up to proteins either on their right or on their left. Later the gene for Anc.3-11 duplicated into Vma3 and Vma11. These new proteins kept doing what the old ones had done: they assembled into rings for pumps. But over millions of generations of fungi, they began to mutate. Some of those mutations took away some of their versatility. Vma11, for example, lost the ability to bind to Vma3 on its clockwise side. Vma3 lost the ability to bind to Vma16 on its clockwise side. These mutations did not kill the yeast, because the proteins could still link together into a ring. They were neutral mutations, in other words. But now the ring had to be more complex because it could form successfully only if all three proteins were present and only if they arranged themselves in one pattern.
Thornton and his colleagues have uncovered precisely the kind of evolutionary episode predicted by the zero-force evolutionary law. Over time, life produced more parts—that is, more ring proteins. And then those extra parts began to diverge from one another. The fungi ended up with a more complex structure than their ancestors had. But it did not happen the way Darwin had imagined, with natural selection favoring a series of intermediate forms. Instead the fungal ring degenerated its way into complexity.
Fixing Mistakes
Gray has found another example of constructive neutral evolution in the way many species edit their genes. When cells need to make a given protein, they transcribe the DNA of its gene into RNA, the single-stranded counterpart of DNA, and then use special enzymes to replace certain RNA building blocks (called nucleotides) with other ones. RNA editing is essential to many species, including us—the unedited RNA molecules produce proteins that do not work. But there is also something decidedly odd about it. Why don't we just have genes with the correct original sequence, making RNA editing unnecessary?
The scenario that Gray proposes for the evolution of RNA editing goes like this: an enzyme mutates so that it can latch onto RNA and change certain nucleotides. This enzyme does not harm the cell, nor does it help it—at least not at first. Doing no harm, it persists. Later a harmful mutation occurs in a gene. Fortunately, the cell already has the RNA-binding enzyme, which can compensate for this mutation by editing the RNA. It shields the cell from the harm of the mutation, allowing the mutation to get passed down to the next generation and spread throughout the population. The evolution of this RNA-editing enzyme and the mutation it fixed was not driven by natural selection, Gray argues. Instead this extra layer of complexity evolved on its own—“neutrally.” Then, once it became widespread, there was no way to get rid of it.
David Speijer, a biochemist at the University of Amsterdam, thinks that Gray and his colleagues have done biology a service with the idea of constructive neutral evolution, especially by challenging the notion that all complexity must be adaptive.But Speijer worries they may be pushing their argument too hard in some cases. On one hand, he thinks that the fungus pumps are a good example of constructive neutral evolution. “Everybody in their right mind would totally agree with it,” he says. In other cases, such as RNA editing, scientists should not, in his view, dismiss the possibility that natural selection was at work, even if the complexity seems useless.
Gray, McShea and Brandon acknowledge the important role of natural selection in the rise of the complexity that surrounds us, from the biochemistry that builds a feather to the photosynthetic factories inside the leaves of trees. Yet they hope their research will coax other biologists to think beyond natural selection and to see the possibility that random mutation can fuel the evolution of complexity on its own. “We don't dismiss adaptation at all as part of that,” Gray says. “We just don't think it explains everything.”
This article was produced in collaboration with Quanta Magazine, an editorially independent division of SimonsFoundation.org.
No comments:
Post a Comment